The discovery of DNA: the molecule of life
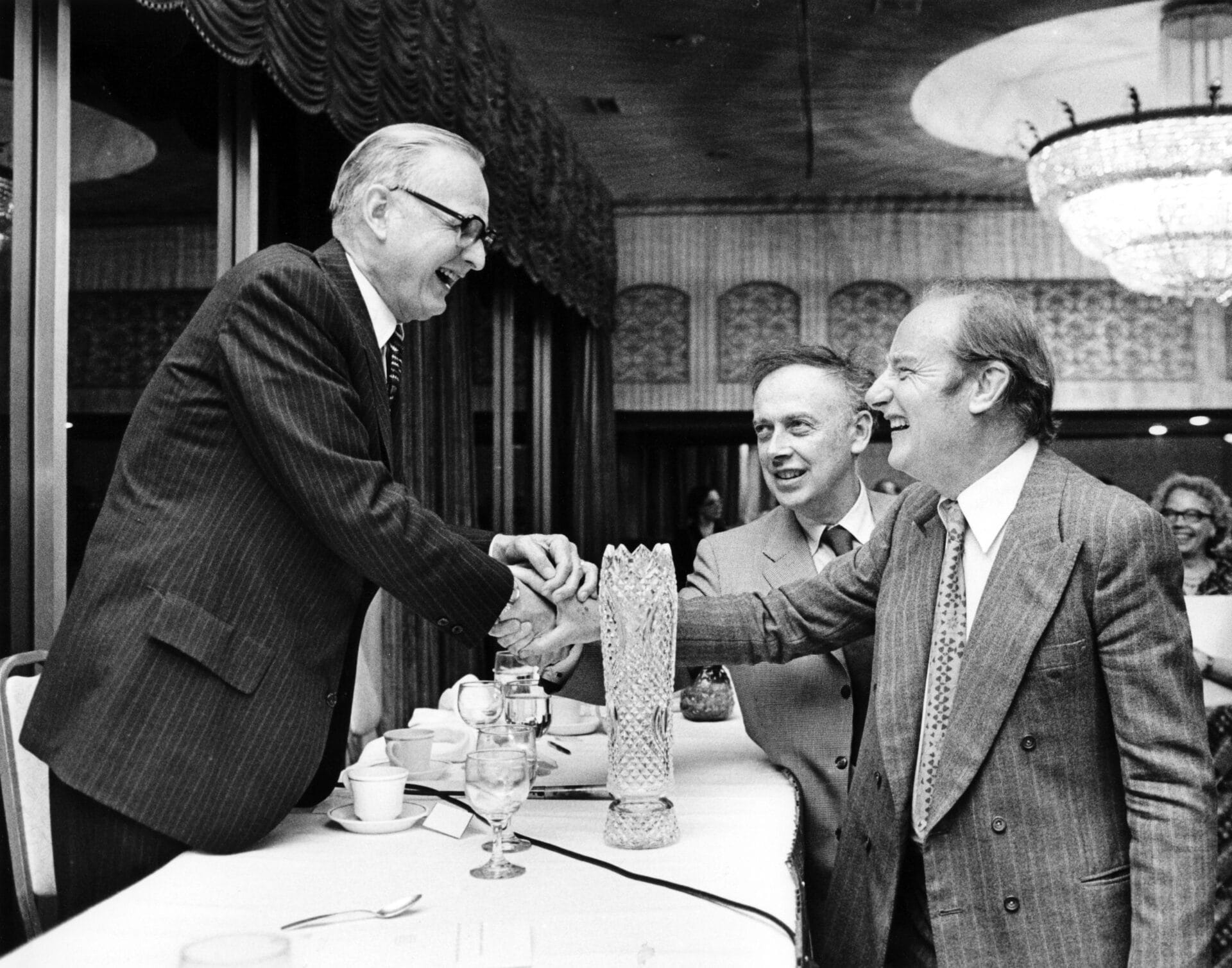
The discovery of DNA marked a milestone in our understanding of the natural world. In the mid 1900s scientists found important evidence on how DNA links to inheritance.
This is part 2 of our series looking at how DNA was discovered and the key players that pushed our understanding forward. To go to part 1 of this series, exploring the first discovery of DNA and the chemicals that make it up, click here.
- It is a common misconception that James Watson and Francis Crick discovered DNA in the 1950s. In reality, DNA was discovered decades before.
- It was by following the work of the pioneers before them that James and Francis were able to come to their ground-breaking conclusion about the structure of DNA in 1953.
- From the mid 1800s to the first decade of the 1900s scientists had made important early discoveries – first discovering and isolating DNA and chromosomes, and then linking making a theory of how chromosomes are linked to inherited characteristics. But the precise mechanics of inheritance were still unclear, and DNA was thought too simple a chemical to play a part.
DNA
(deoxyribonucleic acid) A molecule that carries the genetic information necessary to build and maintain an organism.
Chromosome
A long molecule which stores all or part of the DNA of an organism.
Bacteria
A group of microscopic, prokaryotic, single-celled organisms.
Virus
A group of small infectious agents that can only replicate inside the living cells of another organism.
For the first half of the 20th century, scientists continued to believe that the proteins in chromosomes formed the basis of the genetic information that was passed from generation to generation. To them, DNA was too simple a molecule to be able to carry that sort of complex information and proteins showed much more variation.
However, a series of experiments conducted by various groups of scientists started to reveal that in fact it was DNA, not protein, that carries the genetic information.
Avery-Macleod-McCarty experiment
In 1944, Oswald Avery, Colin MacLeod and Maclyn McCarty helped demonstrate the role of DNA as the carrier of genetic information by working with the bacterium that causes pneumonia, Streptococcus pneumoniae.
However, their work was given a head start by a British bacteriologist called Frederick Griffith, who identified something called the ‘transforming principle’.
Frederick studied two strains of the Streptococcus pneumoniae bacteria. One, called the S strain, had smooth walls and was fatal when injected into mice. The second strain, R, had rough walls and was not fatal when injected into mice. The S strain was smooth due to a coat made out of sugars that helped protect it from the mouse immune system. The rough R bacteria were rough because it did not have a sugar coat, and so was not protected from the mouse immune system.
Frederick carried out a series of experiments to investigate the strains further.
- First he killed S bacteria with heat and injected them into the mice. The mice survived.
- He then injected heat-killed S bacteria along with living R bacteria. The mice died.
- After studying the blood of these mice he was surprised to find living S bacteria in it, somehow the rough R bacteria had transformed into smooth S bacteria.
- He then came to the conclusion that there was a ‘transforming principle’ responsible for this.
But what exactly was it? Was it the proteins in the bacteria, the sugar coat on the S bacteria, the immune system of the mouse or the nucleic acids RNA and DNA?
Enter Oswald Avery and his colleagues. Working in test tubes, they used detergent to break open the heat-killed S cells to separate out the different components:
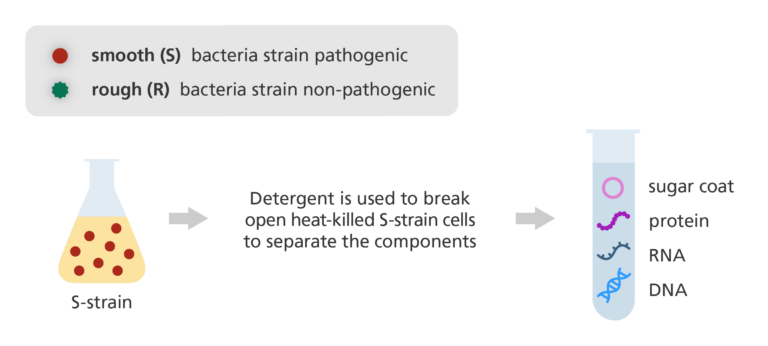
Identifying the ‘transforming principle’
They then destroyed the components one by one to identify which component was the ‘transforming principle’. The team published their results in 1944.
First they combined the heat-killed S bacteria with an enzyme that broke down the smooth sugar coat. They then mixed the sugar-coatless S bacteria with the R bacteria and found that the R bacteria still transformed into S bacteria. So, the ‘transforming principle’ was not in the sugar coat.
Next they added protein-digesting enzymes to destroy all of the protein in the bacteria, and yet again when mixed with R bacteria, the R transformed into S. So the ‘transforming principle’ clearly wasn’t a protein either.
Next they isolated the nucleic acids, DNA and RNA, using alcohol.They then destroyed the RNA using the RNase enzyme, leaving just the DNA behind. They mixed it with the R bacteria, and transformation from R to S still occurred. So, it wasn’t RNA.
Finally, they destroyed the DNA in the solution using DNase, mixed it with the R bacteria, and no transformation occurred, the R bacteria remained rough. So, the ‘transforming principle’ must be DNA!
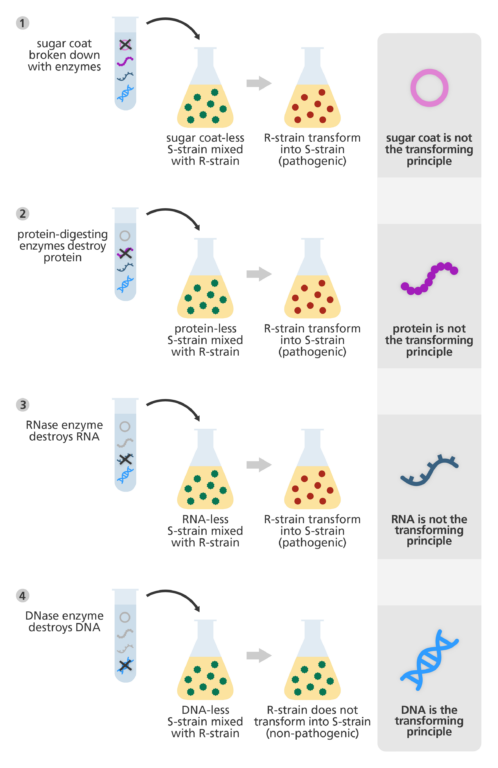
The Hershey-Chase experiments
In 1952, experiments by Alfred Hershey and Martha Chase further supported the findings from the work of Avery, Macleod and McCarty. Hershey and Chase used a virus called T2 to investigate if the genetic information was passed on via DNA or proteins. Although T2 is made up of only a shred of DNA and a scrap of protein, the virus can hijack bacterial cells to make more copies of itself. Scientists knew that the instructions for making new viruses must therefore have been carried in the DNA or protein, but they didn’t know which.
When Hershey and Chase added a radioactive label to the DNA of the original T2 virus, they found that the viruses produced were also radioactive. However, when they repeated the experiment labelling the protein rather than the DNA of the original virus, they found that the viruses produced were not radioactive.
Hershey and Chase concluded that DNA carried the instructions to make new viruses, which was passed on to subsequent generations.
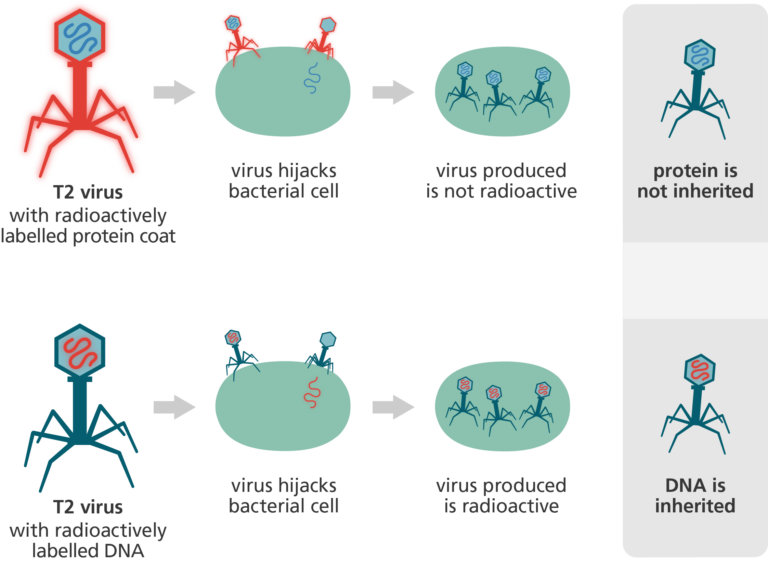
Chargaff and his ‘rules’
The results of experiments by an Austrian scientist Erwin Chargaff helped pave the way to understanding the three-dimensional structure of the DNA molecule.
Erwin set out to investigate if there were any key differences between the DNA of different species. Following this he came to two conclusions, which became known as ‘Chargaff’s rules’:
- In DNA, regardless of which organism it comes from, the amount of adenine (A) is usually the same as the amount of thymine (T), and the amount of guanine (G) is usually the same as the amount of cytosine (C).
- The composition of DNA varies between different species such that the amount of each base is different. This diversity in the composition of DNA made it a much more credible candidate for the genetic material than protein.
Erwin Chargaff’s rules were a crucial step in understanding the structure of DNA. In 1952, he met with James Watson and Francis Crick in Cambridge and discussed his findings with them. Although not the most cordial of encounters, Chargaff’s rules did help James and Francis to explain the three-dimensional structure of the DNA.
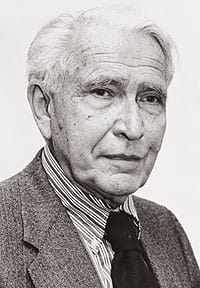