How is genomics being used to tackle schistosomiasis?
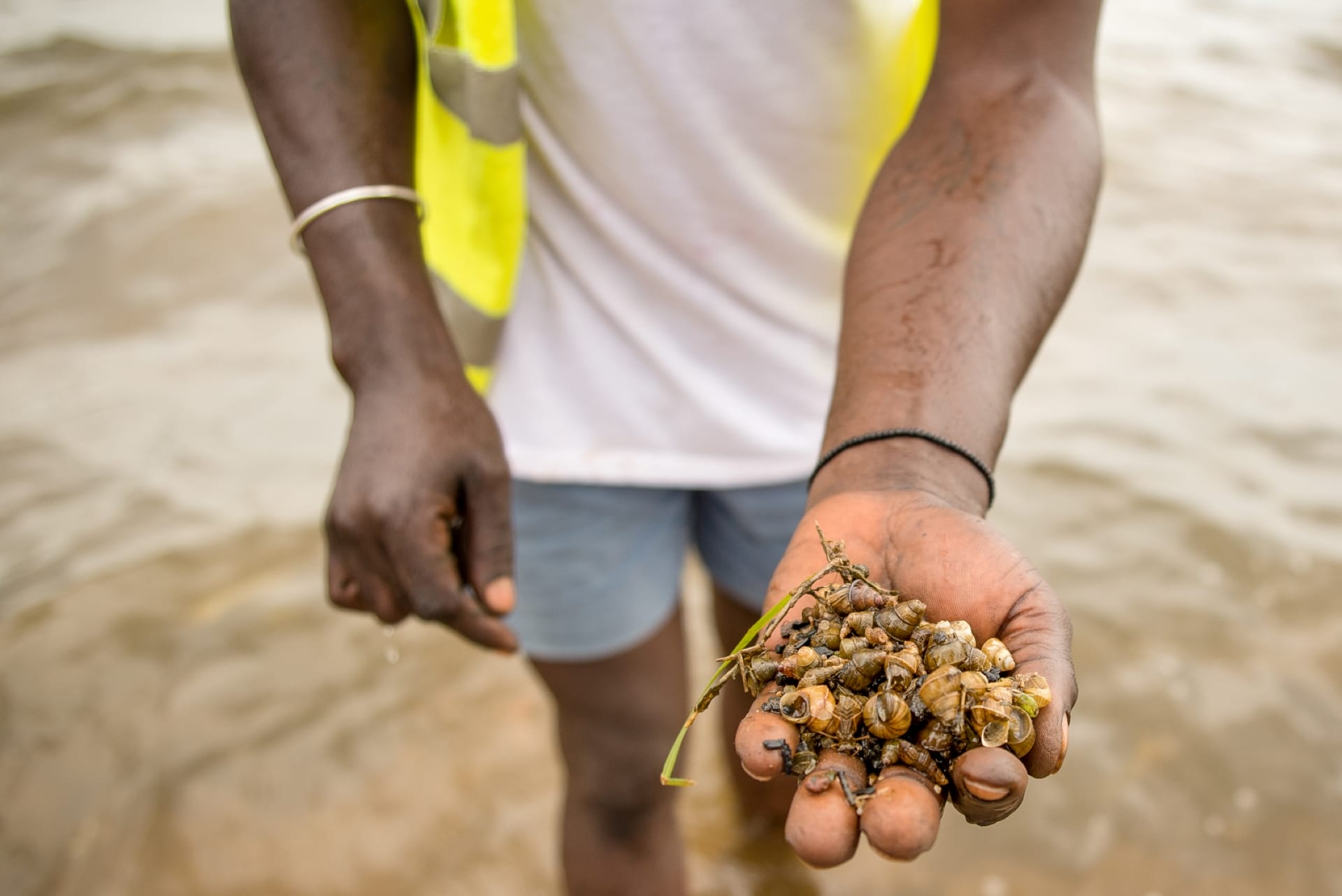
Schistosomiasis is a neglected tropical disease caused by a parasitic worm. It’s transmitted through contact with fresh water that has been contaminated with the parasite’s larvae.
This is part 2 in our series looking at how genomics is being used to tackle neglected tropical diseases. You might want to read part 1 first, here.
- The World Health Organization (WHO) classifies 20 conditions as a ‘neglected tropical disease’, including leprosy, African sleeping sickness and rabies.
- Schistosomiasis is one of these diseases. It affects around 240 million people worldwide and more than 700 million people live in areas where it’s considered ‘endemic’ (or very common).
- Schistosomiasis is most found in communities without clean water, adequate sanitation, or readily available medical treatment.
Key terms
Parasite
An organism that lives in or on another organism (the host) and benefits at the expense of their host.
Model organism
A species that has been widely studied in biology, usually because it is easy to maintain and breed in a laboratory setting and has particular experimental advantages.
What is schistosomiasis?
Schistosomiasis is a neglected tropical disease caused by a group of parasitic worms called schistosomes or Schistosoma. Schistosomes are transmitted by snails that live in fresh water, such as rivers and lakes, in subtropical and tropical regions of the world.
People become infected with the larval form of the parasite, often when washing or playing in contaminated water in lakes and rivers. Chronic symptoms are caused by schistosome eggs becoming trapped in the tissues and organs of the host causing inflammation and scarring. You can read more about the disease in our fact page, here.
Three species of Schistosoma most commonly cause schistosomiasis in humans: S haematobium, S mansoni and S japonicum. S mansoni is the most common, while S hamatobium causes the most dangerous infections.
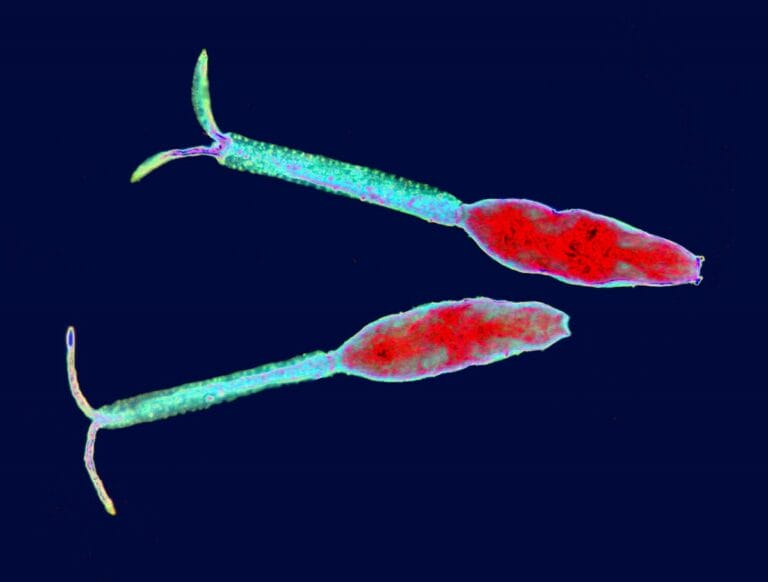
Tiny worm, big genome: sequencing the Schistosoma genome
Despite being a tiny parasitic worm, schistosomes have a surprisingly big genome – about 3.6 million letters long, making it one-tenth the size of the human genome.
But while the human genome was sequenced through an international research project that cost $3 billion, the schistosome genome was worked on by a much smaller team of scientists – making it a much slower, painstaking process.
The first draft of a Schistosoma genome (S mansoni, one of the major schistosome species that cause the disease) was published in 2009, with higher quality genomes of two other schistosome species (S japonicum and S haematobium) published in 2019.
These high-quality genome sequences are helping scientists better understand the parasites and work towards vaccine development.
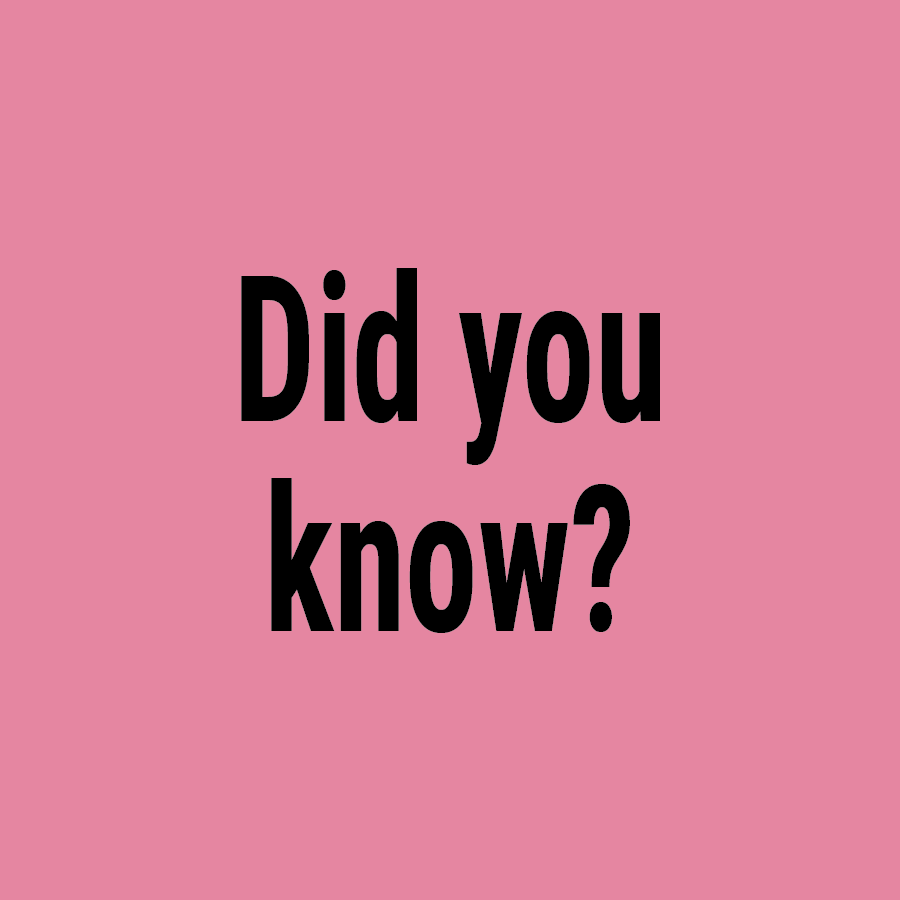
A valuable step in understanding how to tackle neglected tropical diseases is to understand the genomes of the parasites that cause the disease.
Daily rhythms of gene expression
Just as humans have daily rhythms – such as being awake in the morning and sleepy in the evening – so too do other organisms. In most animals, this correlates with the night and day cycle, and affects many processes in the body – including the activity and even the way the blood clots.
In 2021, scientists discovered that Schistosome worms also have daily rhythms. Certain genes are turned on and off across the 24 hours of the day, affecting the worm’s activity.
These changes in gene expression also align with the daily rhythm of the host’s body – which is likely crucial for helping the parasite survive. For example, when the host is active, the parasite turns on genes to protect it from a rise in body temperature. When the host is resting, the parasite turns on genes that help it to produce energy for later. The scientists also discovered that genes involved in reproduction in the worm fluctuate across the day, aligning with daily patterns of laying eggs.
These genetic fluctuations all provide important clues as to how the parasitic worm functions. Tracking gene expression and the underpinning mechanisms could help to design new vaccines and treatments against the Schistosome worm – and even ensure they are delivered at the time of day when they’re most likely to be effective.
Understanding the schistosome life cycle
A great deal of work is focused on investigating the various stages of the schistosome life cycle. Finding a way to interfere with the life cycle of the parasite in the host could help us stop the parasite from causing disease altogether.
This approach has been explored with other parasitic diseases such as malaria – which has now led to two effective vaccines against the parasite that causes severe malaria, Plasmodium falciparum.
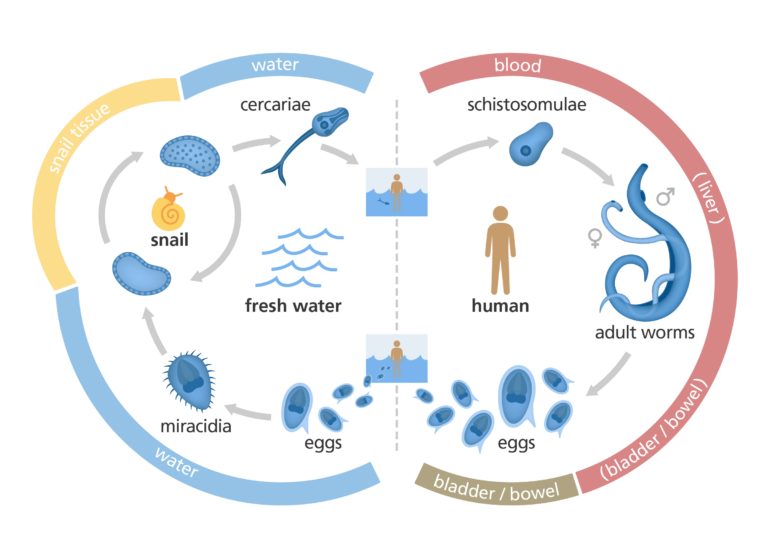
Replicating the schistosome life cycle in the lab
It’s now possible to replicate the life cycle of S mansoni in the lab. This is incredibly useful because it ensures there’s a constant supply of parasites for scientists to investigate.
To maintain S mansoni in the laboratory, scientists keep the parasite’s intermediate vector (a snail) in tropical conditions of around 28˚C. The snails are then ‘shedded’, which involves placing them under UV light to encourage the schistosome larvae out of the snail into the surrounding water. The larvae can then infect a model organism, such as a mouse. Inside the mouse, the schistosome larvae continue their life cycle and can be studied by scientists at each stage.
It is unusual to be able to take a human-infecting parasite and put it in another animal, so it is a huge advantage for studying schistosomiasis that S mansoni infects mice. The only limitation is that in humans, schistosome parasites can stay in the body for 10 years or more. Mice don’t live this long, so it is not possible to study the long term effects of the parasite.
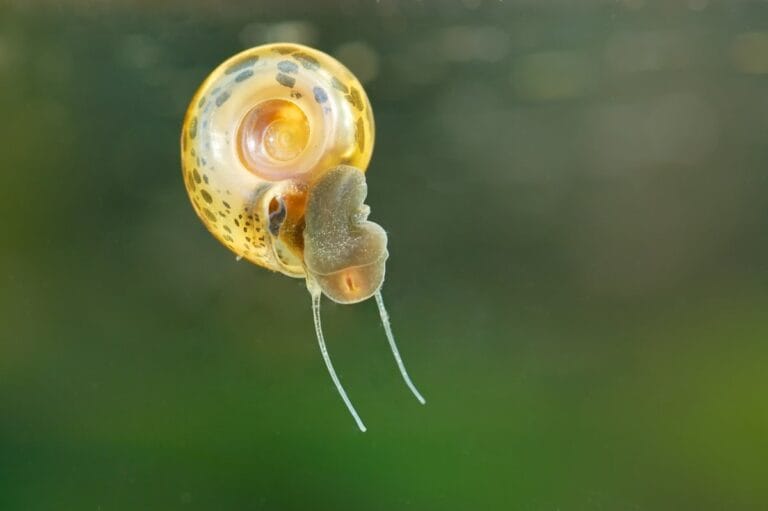
Understanding the metamorphosis stage
When the schistosome larvae burrow through the skin of their new host, they undergo a dramatic transformation, ready for the next part of their life cycle. Later, the parasite journeys through the bloodstream to the liver. Along the way, the parasite transforms again, into a long, thin worm.
By studying the genes that are expressed at these points in the life cycle, scientists can pin down which genes are essential for this transformation – which could lead to new treatments to block their transformation.
Additionally, scientists are studying whether there are any signals that help the parasite find its way to the liver. For example, does the parasite sense something in the host that helps it find its way? Are any of the developmental stages in the worm triggered by knowing it’s in the liver, or knowing it’s in the gut?
Understanding this journey could lead to new ways to prevent the S mansoni parasite from travelling through the bloodstream to infect the liver.
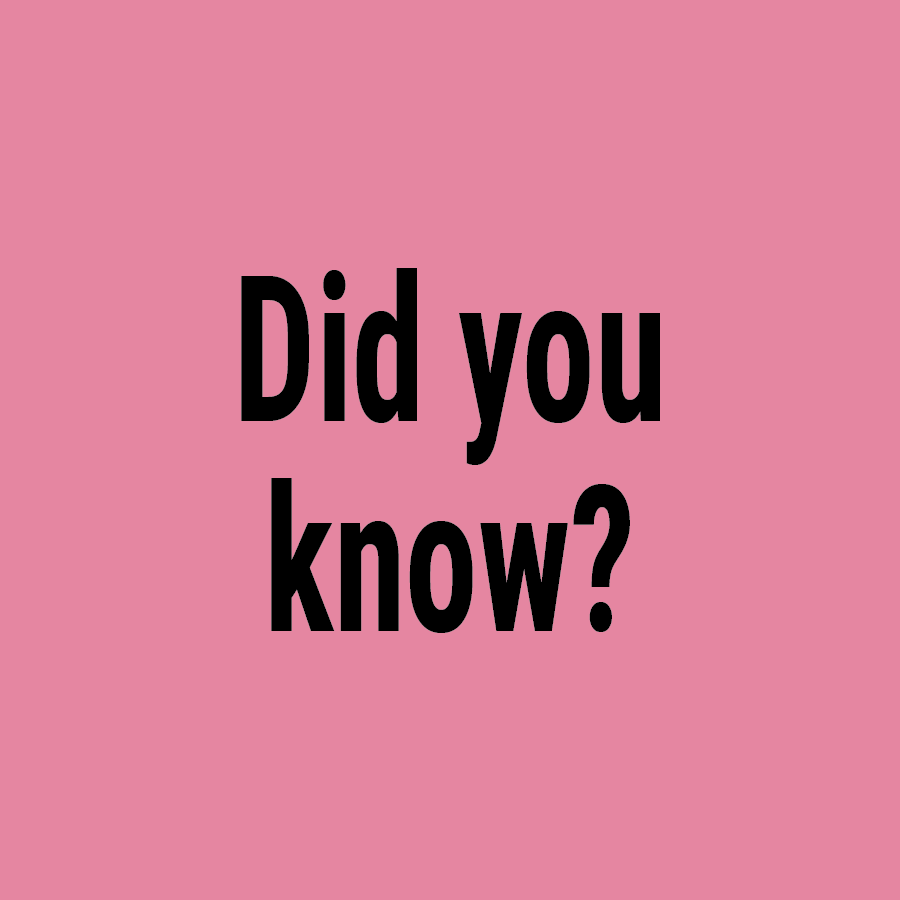
When the schistosome larvae burrow through the skin they undergo a dramatic transformation.
Understanding Schistosome reproduction
Scientists are also interested in how the male and female schistosome worms differ and communicate with each other.
For most of their adult lives, male and female schistosomes are physically bound together so that they can breed. Without the male present the female regresses to a less mature form – but we don’t yet know how this process works.
If scientists can work out how to interfere with breeding in adult worms, they could potentially halt the life cycle altogether. By identifying the genes that are crucial to the growth and development of the schistosome parasite, scientists hope to be able to target them with drugs or vaccines.
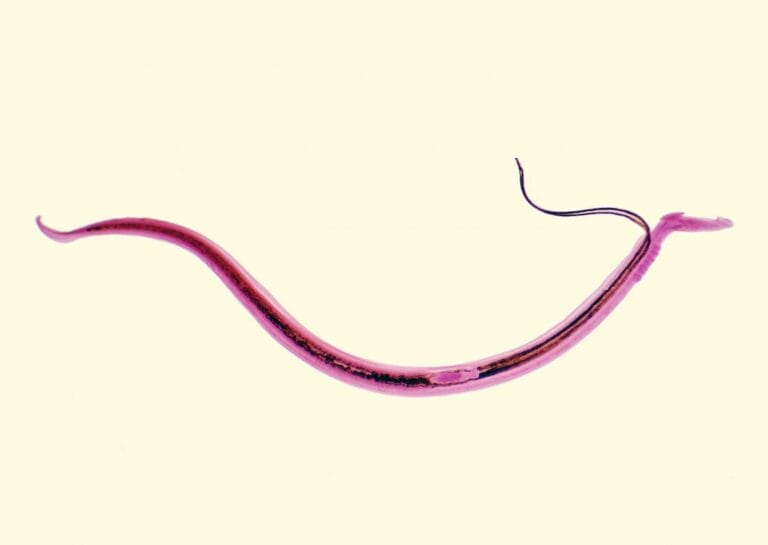